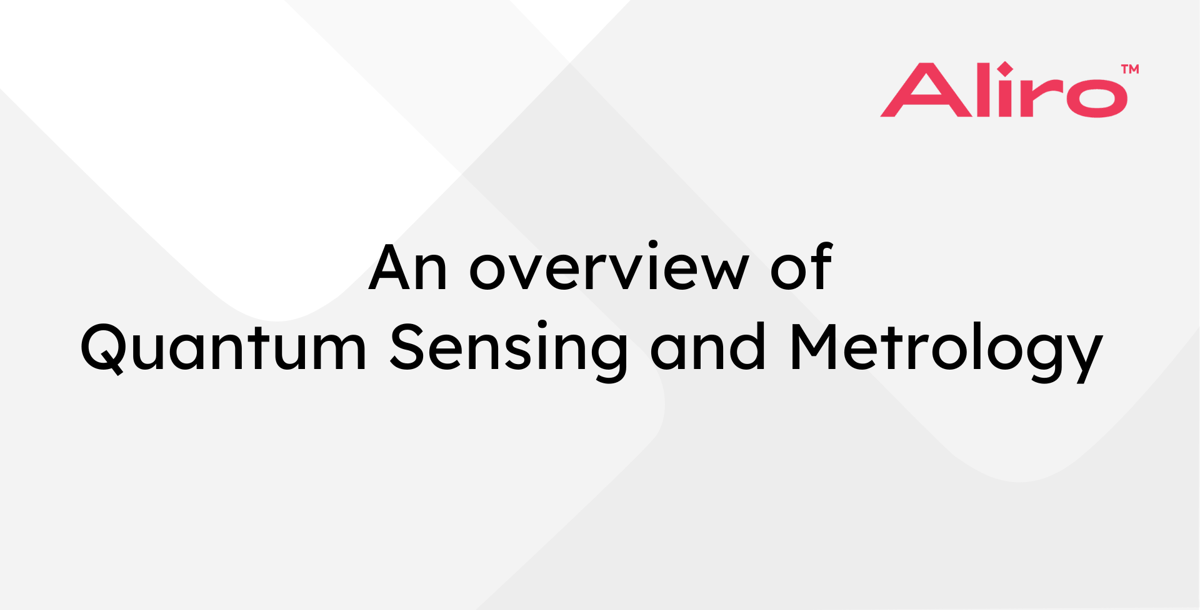
From ultra-precise navigation to exploring cosmic mysteries, quantum sensing is expanding our capabilities in detecting and measuring signals far beyond classical sensing technologies.
Quantum sensors out-perform classical sensors because quantum systems are extremely sensitive to their environment. Leveraging this sensitivity, quantum sensors measure magnetic fields, gravity, time, and more with a very high level of precision. While quantum sensing may seem futuristic, it’s already being used to make precision measurements in science and industry:
- Atomic and Optical Clocks. Atomic clocks measure time with a very high precision using atoms as a reference oscillator. These clocks are foundational to global positioning systems (GPS), telecommunications systems, and financial trading systems. Atoms have stable, well-defined resonant frequencies that can be used to keep time with a high precision. More recently, optical clocks have emerged that can keep time with even higher stability and accuracy.
- Quantum Magnetometers. Superconducting quantum interference devices (SQUIDs) and atomic spin systems can measure very small changes in magnetic fields. Industry applications of magnetometers include geology, material science, and navigation in GPS-denied environments.
- Squeezed Light. Squeezed light sensing is an optical technique in which the sensitivity of one measurement is improved at the expense of another. This technique is applied in the Laser Interferometer Gravitational-Wave Observatory (LIGO), to boost the sensitivity of the gravitational wave detection, leading to more frequent and more precise observations of cosmic events [Aasi2013].
There are two key areas of innovation that will dramatically impact the use of quantum sensors in industries: quantum sensing networks and the miniaturization of quantum sensing devices.
Quantum networks enable entanglement to be distributed across distant quantum sensors. When multiple sensors share entangled states, they can coordinate their measurements in a way that surpasses classical sensing technologies. For instance, entanglement can reduce the error in clock synchronization[Nichol2022] or entanglement can enable long-baseline interferometry in telescope arrays.[Wang2023] As entanglement-based networks are deployed and scaled, they will support networks of quantum sensors that power next-generation global positioning systems and astronomical observatories.
Historically, there has been a trade-off between the size and sensitivity of quantum sensors. As sensing technologies become more sensitive to their environments, they tend to have a larger physical footprint. This poses a challenge to deploying quantum sensors in applications where size and portability are critical, such as in space, mobile, or wearable devices. Despite this challenge, as quantum sensing technologies mature, they are expected to become smaller in size without sacrificing performance.
Quantum sensing technologies will continue to integrate into broader scientific and commercial applications. From pinpointing a location in a GPS-denied environment to uncovering cosmic mysteries, quantum sensors are heralding a new era of discovery.
Curious to Learn More? Aliro has a collection of white papers and reports on quantum networks and entanglement-based solutions that you can download here. https://www.aliroquantum.com/quantum-networking-education
For insights into quantum sensing standards and emerging use cases, check out the Quantum Economic Development Consortium (QED-C) report on quantum sensing. https://quantumconsortium.org/pnt2024/
References
[Aasi2013] Aasi, J. "Enhanced sensitivity of the LIGO gravitational wave detector by using squeezed states of light." Nature Photonics 7 (2013).
[Nichol2022] Nichol, Bethan C., et al. "An elementary quantum network of entangled optical atomic clocks." Nature 609.7928 (2022).
[Wang2023] Wang, Yunkai, Yujie Zhang, and Virginia O. Lorenz. "Astronomical interferometry using continuous variable quantum teleportation." arXiv preprint arXiv:2308.12851 (2023).
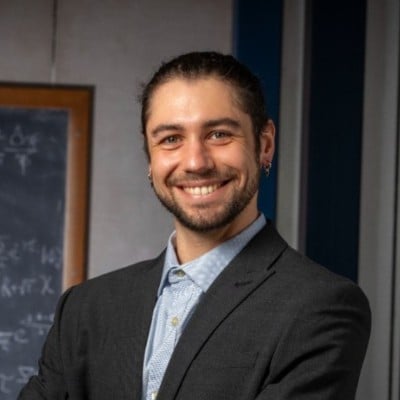