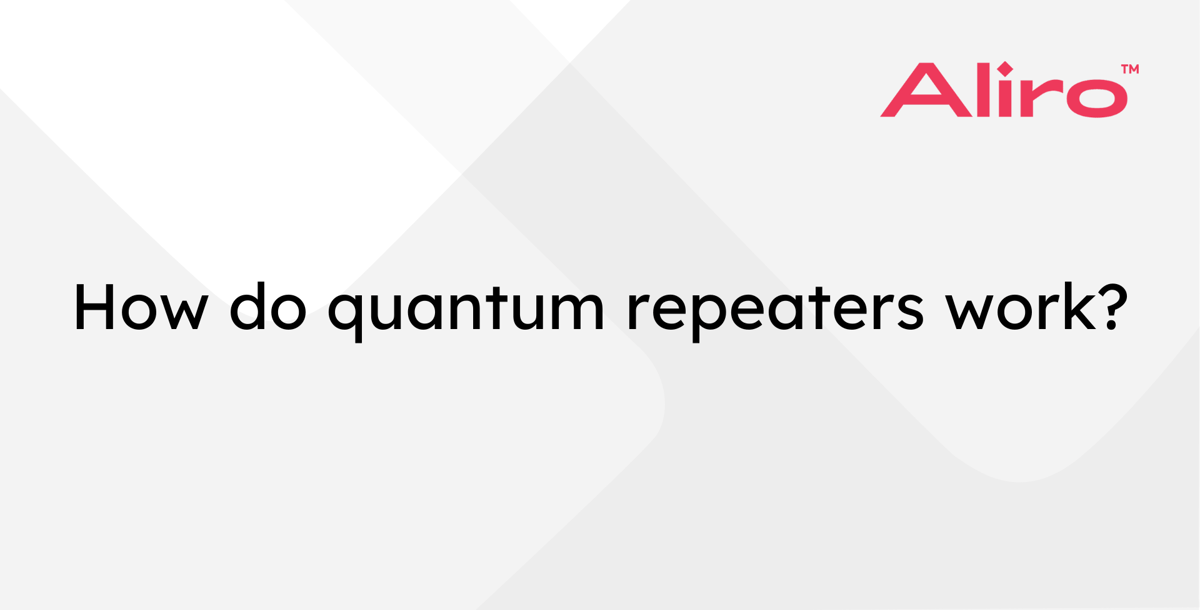
Quantum networks are already being built using the same fiber optic connections used for today's telecommunications infrastructure. However, quantum states are much more fragile than classical ones and need special protections from noise. In order to build quantum networks over long distances, we need a way to combat the noise that disrupts the fragile quantum states stored in photons. Quantum repeaters offer a way to establish entanglement over long distances without sacrificing quality.
Problem: communicating quantum states with noise
Quantum communication is noisy. Noisy communication channels are nothing new -- even classical networks need strategies to reduce errors in transmission. However, because of the especially fragile nature of quantum states and the fact that they cannot be copied, the same techniques that have been used for classical networks fall short for quantum networks.
At the moment, the longest quantum communication demonstrations have been limited to hundreds of kilometers for direct links. Satellite links are able to achieve longer distances; while satellites may well play a role in connecting local networks, they don't replace the need for long-distance fiber transmission. To build a global quantum network, we will need to ability to extend terrestrial links to much longer distances. However, we are fundamentally limited by the fact that noise on quantum channels increases exponentially with distance. Luckily, quantum repeaters offer a different approach: rather than trying to build longer and longer connections, we can construct long-distance entanglement from a series of shorter-distance links.
How do quantum repeaters work?
Quantum repeaters create entangled states between remote nodes by combining a series of elementary entanglements on individual links.
Quantum repeaters rely on a process known as entanglement swapping. Entanglement swapping is a process by which a quantum repeater consumes two halves of entangled pairs, producing a single longer-range entangled pair. Entanglement swapping is actually a special case of the teleportation protocol that is used to transmit qubits over quantum networks.
Entanglement heralding
Entanglement swapping is a probabilistic process -- there is a chance it will fail and we will need to try again. A process called "heralding" allows us to detect whether the swap has succeeded. Quantum networks rely on traditional classical connections for coordination in addition to quantum channels. When a quantum repeater records a successful entanglement swap, it needs to send a classical message to other nodes in the chain to record the successful operation. This message might be sent over the classical internet or over a dedicated classical connection.
Quantum memory
When a node sends half of an entangled pair to a quantum repeater, it needs to hold onto its side of the pair until it hears the successful heralding message from the repeater. Even when they're not in transit, quantum states are very fragile. In order to store the state during this waiting period, the node needs to have some form of quantum memory. One of the major challenges facing quantum networks is the development of quantum memories that can maintain the quality of a qubit for a sufficient amount of time while meeting practical requirements, such as integrating with existing telecommunications infrastructure. There is a major research focus today on developing quantum memories that could meet all of these requirements.
Timing requirements
Even with the best quantum memories, fragile quantum states will degrade in quality if left waiting for too long. In order to preserve quality of communication, quantum networks need to rely on highly precise timing. Distant nodes need to coordinate so that they produce entanglement as precisely the right time. In some cases, timing requirements need to be coordinated to the level of picoseconds (10-12 seconds).
Latest advances
Quantum repeater technology has taken major strides just in the last year. Researchers have demonstrated entanglement swapping in the lab and explored new technologies for quantum memories and entanglement generation. The next steps include increasing entanglement generation rates, extending to networks of additional hops, and ultimately building the first practical networks.
If you found this blog post helpful, you might want to check out our other posts or sign up for the Aliro newsletter, the Quantum Connect, in the footer of this page.
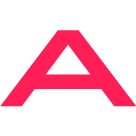