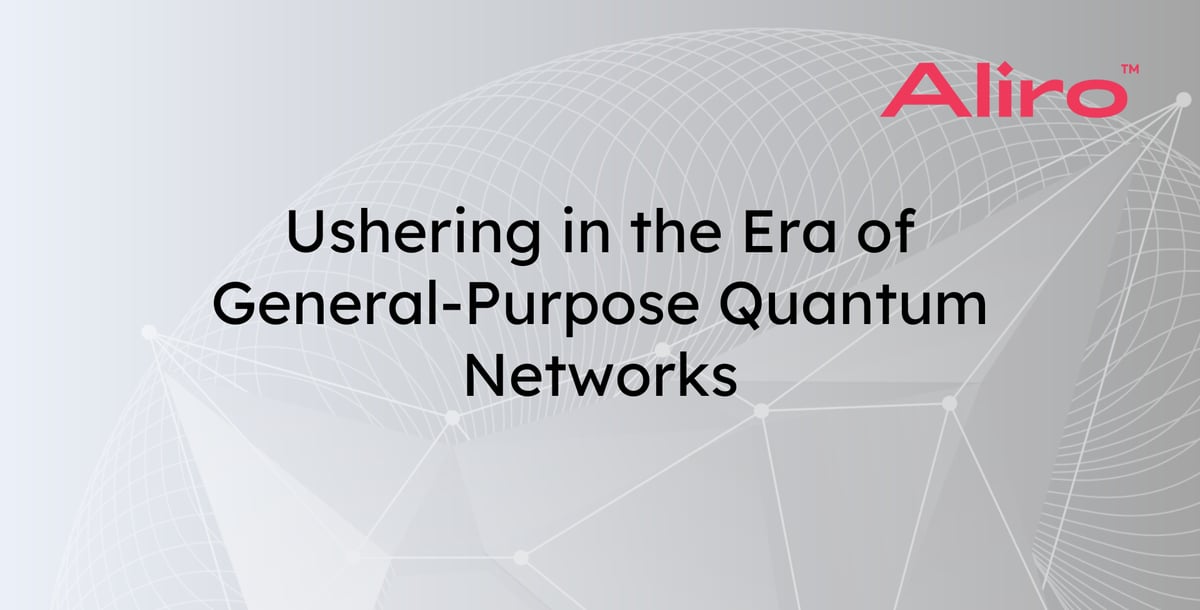
In this article, Aliro CEO Jim Ricotta explains:
- What an entanglement-based Quantum Network is and its transformative potential.
- Why QKD is only the beginning, not the end, of quantum networking solutions.
- The unparalleled security and scalability benefits of entanglement-based Quantum Networks.
This article is based on an interview with Aliro CEO Jim Ricotta, originally conducted by Konstantinos Karagiannis for The Post-Quantum World. You can listen in on the full interview here.
What Is Quantum Networking?
At its core, entanglement-based quantum networking uses entangled pairs of photons to securely transfer information between two nodes. It can be used to generate keys, interconnect quantum computers, and network quantum sensors together. For example, the nodes at either end of a network could be individuals sharing secure messages, or quantum computers connected via the network to scale their capabilities.
In many ways, quantum networks are easier to implement than quantum computing: quantum networks use the same fibers and telecom wavelengths already in place today, and the hardware (like lasers and single-photon detectors) is commercially available. Most of this equipment operates at room temperature making the implementation of quantum networks more feasible than many might assume.
Real-World Applications Are Already Here
Quantum Key Distribution (QKD) has long been seen as one of the first practical applications of quantum networking, using quantum mechanics to securely create shared symmetric encryption keys, protecting them from interception or eavesdropping and addressing vulnerabilities in RSA and Diffie-Hellman protocols. However, this is only the beginning. QKD’s single-purpose nature limits its utility compared to the broader potential of general-purpose quantum networks. While QKD addresses a critical security challenge, the true power of quantum networking lies in its ability to support a wide range of applications, including direct data transmission, quantum computer interconnects, and quantum sensor networks. General-purpose entanglement-based quantum networks are not a single-purpose solution—these networks are more like the TCP/IP of the quantum world.
To build general-purpose quantum networks, Aliro leverages well-established protocols like BBM92 and E91, which are entanglement-based versions of the foundational BB84 protocol used in QKD. These protocols enable entanglement-based communication, allowing the network to not only generate secure keys but also transmit quantum information directly between nodes. For example, BBM92 utilizes entangled photon pairs to securely share information between parties while detecting any potential eavesdropping through quantum bit error rates. Similarly, the E91 protocol enhances security by incorporating quantum correlations to verify shared information between nodes. These protocols make use of entanglement. Any interference or eavesdropping would collapse the quantum state, and users would know something is wrong - too much information is being disrupted to be considered secure.
Today, these protocols are primarily used to generate secure keys. As entanglement rates improve to hundreds of thousands or millions of pairs per second, the quantum channel itself will be used for transmitting messages and files. This shift would eliminate the need for classical channels to carry encrypted data, allowing quantum networks to deliver unprecedented levels of security and efficiency for large-scale communication and computation. The evolution of these protocols underscores the pathway to general-purpose quantum networking and its transformative potential.
Even more exciting is the potential for creating powerful quantum computers through networking QPUs, as with Aliro’s work with the U.S. Air Force in connecting quantum computers of different modalities: a superconducting quantum computer from Rigetti and a trapped-ion system from IonQ. This interconnect capability is crucial for scaling quantum computing, as it allows different types of qubits to collaborate effectively.
To jumpstart this work, AFRL is using the Aliro Simulator. The Aliro Simulator was initially built to enable Aliro’s own product development, but it’s become a powerful stand alone product that organizations like the Air Force use to experiment and refine their quantum network designs. The significance of Aliro Simulator lies in its ability to model quantum networks at varying levels of granularity. At a high level, the simulator can analyze traffic patterns, node configurations, and overall network architecture. At a more detailed level, it incorporates the physics of fiber optics, including loss, dispersion, and the behavior of individual components like lasers and single-photon detectors. The network’s performance can be simulated at that higher level, but the quantum properties of the hardware (such as the dark counts of photon detectors or the reset times required) can also be modeled accurately. This comprehensive modeling capability allows users to predict the behavior of their quantum networks under realistic conditions.
For the Air Force project, simulations are used to model the connection between the superconducting quantum computer from Rigetti and the trapped-ion system from IonQ. This task involves complex transduction processes, converting qubit states into photons for transmission and back into qubit states at the receiving end. Different configurations and protocols can be modeled prior to physically connecting these systems, reducing the risk and cost associated with trial-and-error.
Beyond military applications, simulation is valuable for any organization looking to explore quantum networking. It supports research and development by allowing users to test new protocols, evaluate hardware performance, and model emerging use cases, such as quantum key distribution or quantum sensor networks. Simulation is almost like a quantum testbed in software form. Enterprises, telcos, and government agencies can use it to gain hands-on experience with quantum networking, even if they haven’t deployed the physical hardware yet. Enabling organizations to experiment and learn in a low-risk environment accelerates innovation and helps bridge the gap between theoretical research and real-world deployment. Simulation in this environment is about making quantum networking accessible, scalable, and ready for the challenges of tomorrow.
The EPB Quantum Network
The EPB Quantum Network in Chattanooga, Tennessee, stands as a pioneering example of how quantum networking is already being utilized in the United States. Developed by EPB, this network is the first commercially available quantum network in the country. The EPB Quantum Network uses the same fiber already in place, combined with commercially available hardware like lasers and single-photon detectors, and runs on Aliro’s software. This combination of accessible hardware, pre-existing infrastructure, and scalable software has enabled EPB to offer the network as a testbed for researchers and organizations interested in hands-on experimentation with quantum networking.
What sets the EPB Quantum Network apart is its availability to third parties. Universities, startups, and established enterprises can lease time on the network to test quantum technologies, run experiments, and develop new protocols.This is a great example of how public-private collaboration can drive quantum innovation, as well as position the U.S. as a leader in the global race to develop and deploy quantum networks.
The Future is Quantum Networking
The future of quantum networking is anything but hypothetical. Whether it’s interconnecting quantum computers, securing critical communications, or laying the foundation for a quantum internet, entanglement-based quantum networking is the key to unlocking many other quantum technologies.
Entanglement-based quantum networks are the future of networking. It’s a whole new world, and it’s going to enable things we can’t even imagine yet. For business leaders and technologists, the message is clear: now is the time to get involved in entanglement-based quantum networking.
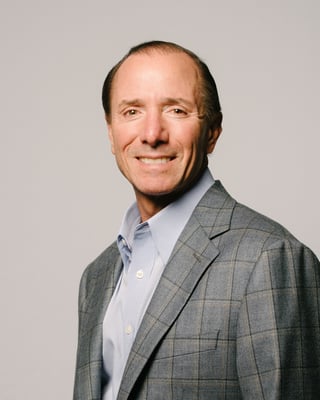